Chapter 25 Section 1 Guided Reading and Review Mobilization
Abstract
Circulating bone marrow (BM)-derived stem and progenitor cells (SPCs) participate in turnover of vascular endothelium and myocardial repair after acute coronary syndromes. Acute myocardial infarction (MI) produces a generalized inflammatory reaction, including mobilization of SPCs, increased local production of chemoattractants in the ischemic myocardium, as well as neural and humoral signals activating the SPC egress from the BM. Several types of circulating BM cells were identified in the peripheral blood, including hematopoietic stem cells, endothelial progenitor cells, mesenchymal stromal cells, circulating angiogenic cells and pluripotent very small embryonic-like cells; however, the contribution of circulating cells to the myocardial and endothelial repair is still unknown. The number and function of these cells is impaired in patients with diabetes and other cardiovascular risk factors, but can be improved by physical exercise and use of statins. The mobilization of SPCs in acute coronary syndromes and stable coronary artery disease seems to predict the clinical outcomes in selected groups of patients. Interpretation of the findings has to incorporate other factors that modulate the process of mobilization, such as coexisting diseases, age and medications. This review discusses the mobilization of SPCs in acute ischemia (MI, stroke), as well as in stable cardiovascular disease, and highlights the possibility of using the SPC as a marker of cardiovascular risk.
Introduction
Stem and progenitor cell (SPC) biology became a common point of interest for cardiovascular (CV) physicians and basic scientists with the ultimate goal of translation into clinical application by use of the innate reparatory mechanisms of the heart and vascular endothelium. Bone-marrow-derived stem cell therapy of the heart became a reality 9 years ago; however, we still do not know the exact mechanisms of physiological response of the bone marrow (BM) compartment evoked by myocardial ischemia. Acute myocardial infarction (MI) produces a generalized inflammatory reaction, a part of which is mobilization of SPCs, increased local production of chemoattractants in the ischemic myocardium, as well as neural and humoral signals activating the stem cell egress from the BM. Numerous studies confirmed that MI induces rapid mobilization of hematopoietic stem cells (HSCs), endothelial progenitor cells (EPCs), mesenchymal stromal cells (MSCs), circulating angiogenic cells and pluripotent very small embryonic-like cells (VSELs); however, the contribution of circulating cells to the myocardial and endothelial repair is still incompletely understood, although experimental studies have suggested an important role of BM-derived stem cells in the cardiac repair response after MI. Acute MI is associated with substantial tissue necrosis, so the signals for SPCs may be regarded as a part of the inflammatory reaction; however, even in healthy subjects and patients with stable coronary artery disease (CAD) there is a constant mobilization of a small number of SPCs, which probably participate in endothelial and cardiac renewal. This review discusses the mobilization of SPCs in acute ischemia (MI, stroke), as well as in stable cardiovascular disease, and highlights the possibility of using the SPC as a marker of cardiovascular risk.1, 2
Mobilization of SPCs in acute coronary syndromes
Heterogeneity of circulating SPCs
Ischemia and myocardial necrosis form a milieu within the BM and cardiac tissue accompanied by increased levels of chemoattractants (bioactive phospholipids, kinins, chemokines, cytokines, growth factors, complement cascade), which promote the release of a heterogenous population of BM cells. This phenomenon was observed many years ago when increased leukocyte count in acute MI was described and was considered to be an unspecific inflammatory reaction. In general, the majority of mobilized cells are committed lineages, including granulocytes, lymphocytes, CD14+ monocytes and a very small number of cells with immature phenotype.1 Many studies that investigated mobilization of BM cells in acute coronary syndromes had a pivotal limitation, which is the use of a single surface marker for identification of the cell types, for example, CD34+, which makes it impossible to directly compare the findings and to form a viable hypothesis on the role of a particular cell population in the reparatory process.3, 4 The methodology of SPC measurement is beyond the scope of this review; however, some important issues that determine the validity of the results have to be considered. In particular, so far there is no consensus on the identification criteria even of the most frequently investigated population of EPCs. Use of less stringent criteria, for example, by employing assays based on a single marker, can overestimate the number of SPCs, which are extremely rare both in the BM and in peripheral blood (PB).4 There is an overlap in expression of surface markers and a set of several markers needs to be employed to identify a particular population of cells.5, 6, 7
Endothelial progenitor cells
Ten years ago, Shintani et al. 3 described for the first time the rapid mobilization of CD34+ cells in acute MI. Cells were further characterized in cultures as EPCs. The number of released cells reached a maximum 7 days after the onset of MI parallel to significant increase of plasma levels of vascular endothelial growth factor (VEGF). Follow-up 1 month later showed that the number of EPCs was still elevated in comparison with baseline. These observations were subsequently confirmed by Massa et al.,8 who showed spontaneous mobilization of EPCs, which reached a maximum very early, ∼3 h after the onset of myocardial ischemia, and decreased to values comparable to healthy subjects within 60 days. After immunomagnetic purification of circulating CD34+ cells it was shown that the level of co-expression of CD133 and VEGFR2 is significantly higher in patients with acute MI, suggesting that increase of EPCs is responsible for the higher number of CD34+ cells. Also, the in vitro study showed an increased number of endothelial colonies and a gradual increase of VEGF levels. Several other studies confirmed the very rapid release of EPCs in acute MI, in contrast to the landmark study of Shintani that suggested delayed mobilization of CD34+ cells. Interestingly, mobilized CD34+ cells expressed higher levels of CXCR4, LFA-1, VLA-4 and ICAM-1 receptors in comparison with patients with stable CAD, which theoretically would promote the homing of these cells to the ischemic area.9 The type of primary treatment of AMI does not seem to affect the mobilization, because a similar pattern of CD34+ cell increase was found in patients who received thrombolytic therapy instead of primary angioplasty.10 Also, an increased number of EPCs was observed for as long as 3 months after the episode of unstable angina, which, in contrast to MI, involves myocardial ischemia but not extensive necrosis, so it is likely that the chemoattractants are more effectively released by viable myocardial tissue than in an acute setting.11 Since the first description of CD34+ EPCs by Asahara et al. 12 in 1997, a variety of studies measured the EPCs in patients with CAD using different protocols.7 So far the data from these studies show that EPSc form a heterogenous population of cells. The most widely accepted phenotype for identification of EPCs by FACS comprises CD34+/KDR+ (kinase insert domain containing receptor) and CD133+/KDR+ cells.5, 13 Also accumulating evidence supports the hypothesis that a population of circulating CD14+ monocytes can be a source of EPCs.14, 15
Moreover, Krankel et al. 16 showed that type B2 receptor for bradykinin is expressed on EPCs. The presence of type B2 receptor is consistent with the chemoattractant effects of bradykinin, which in turn is guided by the migration of proangiogenic progenitor cells. Therefore, inclusion of type B2 receptor into the array of surface markers detected on EPCs might help identify the population of 'angiogenic' EPCs. Currently, modified protocols (for example, the modified International Society of Hematotherapy and Graft Engineering protocol) are developed to more effectively identify the EPCs. The use of this protocol showed that the target population of EPCs is a subpopulation of CD45dim/CD34+/KDR+ cells.17 Only when uniform cell enumeration protocols are used might the results from different laboratories be compared. A major difference between the studies is the use of the FACS-based or culture-based approach of EPC identification. Even the protocols based on cell cultures are non-uniform and results difficult to compare. It seems that interpretation of findings from studies investigating circulating EPCs should be adjusted according to the particular protocol. For example, decrease of EPCs in patients with CAD is consistently reported in studies using either the FACS-based or the culture-based protocols; however, in patients with ACS the increase in EPC number reported in studies using the 'standard' FACS approach (CD34+/KDR+) was not observed when the modified International Society of Hematotherapy and Graft Engineering protocol (CD45dim/CD34+/KDR+) was employed.17 In several studies the protocols based on cell culture were used with or without concomitant identification of EPCs by FACS. So far the most widely used approach for enumeration of EPCs is a FACS-based protocol, which gives an idea about the cell number and allows for correlation with clinical markers and outcomes, but does not necessarily overlap with 'true' angiogenic cells identified by cell culture.7, 18 In addition, the population of circulating progenitor cells can be identified by staining for aldehyde dehydrogenase, which identifies cells expressing CD34 and CD133 markers and correlates with the number of CD133+CD34+ EPCs.19
Last but not the least, circulating EPCs on different stages of maturation should be distinguished from circulating endothelial cells (CECs), which are sloughed from the arterial wall. CECs present mature phenotype (CD34−, CD133−, CD146+, von Willebrand+) and have no potential to form colonies in vitro. CEC number is increased in ACS, stroke and critical limb ischemia in a similar manner as immature SPCs. Probably the endothelial cellular turnover, which can be roughly estimated as the ratio of CEC to EPC, will yield more information on individual reparatory potential than measurement of either single population of cells. The differentiation between EPCs and CEC based just on the flow cytometry might be suboptimal, because of some degree of overlap of the populations expressing 'EPC-specific' and 'CEC-specific' markers.20 The differentiation between populations of circulating SPCs is more effective when identification with FACS is supported by colony-forming unit assays and studies of angiogenic potential. These methods are, however, not compatible with routine clinical practice, such as risk stratification. Indeed, the study of Fadini et al. 21 in 21 patients with metabolic syndrome showed that the number of CD34+ cells is a better predictor of cardiovascular risk than analysis of cell subpopulations (CD133+, CD34+/CD133+, CD34+/KDR+, CD133+/KDR+, and CD34+/CD133+/KDR+). Therefore, in order to use the number of EPCs as a surrogate marker of CV risk, it would be more appropriate to use the simpler approach and choose a surface marker (CD34+), which might not differentiate between particular subsets of cells, but show best correlation with CV risk burden.
Hematopoietic stem cells
Initial studies showing the release of CD34+ in acute coronary syndromes were followed by more detailed cell characterization with FACS protocols consistent with the International Society of Hematotherapy and Graft Engineering protocol guidelines. Massa et al. 8 showed that HSCs are mobilized soon after the onset of acute MI and the release kinetics is similar to EPCs. The populations of cells isolated from PB were CD34+/CD117+, CD34+/CXCR4+, CD34+/CD38+ and CD34+/CD45+. Mobilization of HSC has been confirmed by cell cultures, which revealed increased number of erythroid burst-forming units and granulocyte macrophage colony-forming units soon after onset of acute MI. We have also demonstrated rapid mobilization of HSC-enriched populations of CD34+/CXCR4+ and CD34+/CD117+ stem cells in the setting of acute MI, with maximum cell efflux within the first 12 h after the chest pain and decrease over 1 week.22 Therefore, consistent data confirm that not only EPCs but also HSCs contribute to the pool of SPCs mobilized by acute MI. Conversely, Leone et al. 23 showed that in a substantial number of patients the maximum mobilization of HSCs occurred later, on the fifth day after acute MI. Interestingly, the elevated number of HSCs was not related to increased leukocyte count.8
Stem cells expressing early developmental and tissue specific markers (VSELs)
Our initial studies showed that in patients with acute MI parallel to the mobilization of CD34+/CXCR4+ and CD34+/CD117+ cells there is a marked upregulation of genes coding for specific cardiac (GATA4, MEF2C and Nkx2.5/Csx), muscle (Myf5, Myogenin and MyoD) and endothelial (VE-cadherin and von Willebrand factor) markers in peripheral blood mononuclear cells.22 Following this finding, a small population of CXCR4+ cells displaying the phenotype of primitive pluripotent cells was characterized and named VSELs. We established the protocol of FACS-based live cell sorting, which is most efficient for isolation of this rare population of cells. The detailed protocol and characteristics of VSELs were described elsewhere.24, 25 In brief, VSELs are non-hematopoietic lin−/CD45−/CD133+ cells enriched for early embryonic markers (Oct-4, Nanog, SSEA-4, Rex1, Dppa3 and Rif-1) and expressing CXCR4 receptor. The morphology and size of VSELs resemble those of pluripotent embryonic stem cells and are markedly different from and significantly smaller than HSCs (open-type chromatin, large nucleus, narrow rim of cytoplasm with multiple mitochondria; 3–6 μm vs 6–8 μm).25, 26 In healthy adult humans PB contains a very low number of VSELs, ∼1–1.5 cells/μl. In the setting of acute MI, VSELs undergo rapid mobilization within 12 h similarly to HSCs and EPCs, with the exception that the absolute number of cells is lower than that for the other two populations. Release of VSELs into PB coexists with marked increase of the expression levels of mRNA of PSC markers (Oct-4, Nanog), as well as early cardiac (GATA-4, Nkx2.5/Csx and MEF2C) and endothelial markers (VE-cadherin).27 The primitive phenotype of these cells and ability of murine VSELs to undergo expansion and differentiation into cells from three germ layers including cardiomyocytes raises the exciting possibility that they constitute a population with high reparatory potential. VSELs express chemokine receptor CXCR4, so they can react to the homing signals produced by ischemic myocardial tissue.28
Mesenchymal stem cells
Mesenchymal stem cells might potentially be optimal cells for cardiac repair, given their immunoprivileged status. It seems most probable that MSCs are mobilized into peripheral blood along with other populations of BM cells. However, the data regarding the kinetics of MSCs mobilization are not consistent and need confirmation.29 A recent study of Iso et al. 30 identified a population of CD271+ MSCs released to the PB in acute MI reaching a peak number after 3 days and returning to levels comparable to healthy subjects by 1 week.
Other acute cardiovascular events associated with mobilization of SPCs
Stroke
Cerebral ischemia leads to the mobilization of several populations of CD34+ cells, including HSCs and CXCR4+lin–CD45– VSELs expressing pluripotent markers (Oct-4, Nanog) and neural-specific markers (GFAP, nestin, βIII-tubulin, Olig1, Olig2, Sox2 and Musashi). The number of cells was positively correlated with stroke extensiveness. Increased numbers of HSCs and VSELs were observed 24 h after stroke and lasted for at least 7 days.31 On the other hand, the number of EPCs measured as CFU and their ability to form tubes in matrigel assay were reduced in acute stroke. The number of cells was measured, however, ∼1 week after the stroke, which might be too late to measure the 'true' reparative cells.32
Limb ischemia
EPC mobilization can be triggered by a single episode of limb ischemia induced by transient tourniquet occlusion of blood flow to one lower extremity in healthy volunteers, suggesting that this phenomenon is a fast-acting universal response to large-tissue ischemia.33
Cardiac non-ischemic injury
Several clinical scenarios associated with major ischemia can lead to rapid release of SPC from the BM. Besides acute MI, another form of myocardial injury is the radiofrequency catheter ablation of atrial fibrillation, which was shown to induce the mobilization of CD34+ and was correlated with the extent of myocardial necrosis; however, this finding was not confirmed in other similar studies.34, 35
In general, consistent findings suggest that substantial ischemia evokes a transient release of SPC from the BM, including populations with capacity of endothelial differentiation and induction of angiogenesis. It seems that the ischemia is a prerequisite for significant mobilization of EPCs, because, as shown by Adams et al.,36 the EPC number increased only in patients with inducible myocardial ischemia, but not in healthy subjects or patients without inducible ischemia. Also, in the hearts explanted from patients with chronic ischemic cardiomyopathy, the expression of chemoattractants (stromal cell-derived factor-1 (SDF-1), stem cell factor, hypoxia-inducible factor-1α, hepatocyte growth factor) was increased in comparison with non-ischemic cardiomyopathy (dilated cardiomyopathy). The number of circulating cells in ischemic cardiomyopathy was lower than in dilated cardiomyopathy, perhaps as a result of more effective homing signals.37
Mechanisms of mobilization in acute cardiovascular events
In acute MI, the systemic levels of soluble inflammatory mediators are significantly increased. Among inflammatory markers there are numerous factors that act as chemoattractants to BM SPC. It seemed appealing to assume that ischemic tissue releases the chemoattractants, which create the gradient directing to the site of injury and that the cells follow the migratory pathway created by increased levels of chemokines, cytokines and growth factors.1, 38 The true mechanism is, however, more complicated, because, first, the plasma levels of chemokines (SDF-1) are not correlated with the extent of SPC mobilization; second, the measurement of plasma levels does not necessarily reflect the local concentrations in the BM and ischemic tissue, which is more important for cell egress and homing. Third, until recently factors more important for SPC mobilization than circulating chemokines were unknown, such as bioactive phospholipids (sphingosine-1-phosphate, activation of complement cascade and kinin–kallikrein system, including presence of bradykinin B2 receptor on circulating EPCs).16, 39, 40
In general, the studies in patients with acute MI showed either weak correlation between the number of EPCs and plasma VEGF levels, and CXCR4+ cells and plasma SDF-1 levels, or found no significant correlation at all. Most likely the timing of the blood sampling is crucial, because the early kinetics of SPC mobilization does not entirely overlap with prolonged inflammatory reaction.3, 8, 22, 23 Several chemoattractants are significantly upregulated in the viable ischemic area of the myocardium surrounding the necrotic infarct zone.41 Moreover, the significant correlations do not necessarily prove the causal link between the SPC number and soluble cytokine levels, due to the complexity of inflammatory reaction in ACS. Circulating SPCs express several receptors for cytokines, growth factors and chemokines. Data from animal and human studies suggest that the local milieu created in the infarct border zone may attract the circulating cells via several signaling pathways, including membrane receptors CXCR4 and CXCR7, interacting with chemokine SDF-1. CXCR4+ cells enriched for cardiac and endothelial markers show robust chemotactic response to an SDF-1 gradient and expression of SDF-1 in ischemic myocardium was shown both in murine models and in human tissues. This chemokine has a pivotal role in the retention and mobilization of CXCR4+ SPC from the BM, and their homing and engraftment in the myocardium is basically a reverse process to mobilization. Other signaling pathways that might promote trafficking and engraftment of SPC are leukemia inhibitory factor– leukemia inhibitory factor receptor, hepatocyte growth factor–c-met, VEGF–VEGFR and stem cell factor–CD117. All of them are upregulated in acute MI.38, 42 Figure 1 shows the mechanisms involved in mobilization of BM cells in acute MI and vascular endothelial injury (rupture of unstable plaque and stent implantation), and homing of SPCs to the site of injury.
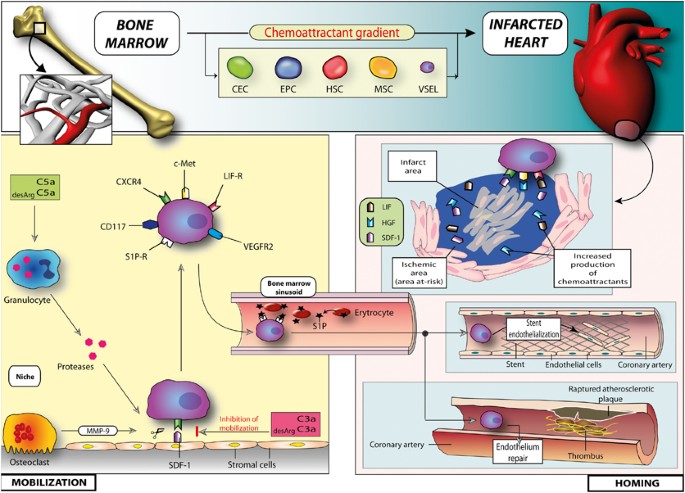
Mechanisms involved in mobilization of BM cells in acute MI and vascular endothelial injury (rupture of unstable plaque and stent implantation), and homing of SPCs to the site of injury.
Full size image
Factors modulating mobilization of SPC
The absolute number of SPCs in PB is very low even in the setting of acute cardiovascular events, and their ability to engraft in the ischemic area is definitely limited, as shown in studies with intracoronary infusion of radiolabelled cells. Therefore, to properly interpret the differences in the numbers of circulating SPCs, it is important to account for other factors that can modulate this process, including age, medications, profile of CV factors and major vascular surgical procedures. In general, the number and function of circulating cells, particularly EPCs, is reduced in older subjects with coexisting cardiovascular risk factors, especially diabetes. Diabetes is associated with BM dysfunction, most probably associated with generation of reactive oxygen species, which translates into structural disorganization of vascular niche and dysfunction of BM MNCs.43 The number of circulating EPCs declines proportionally to the increasing number of cardiovascular risk factors (Tables 1 and 2). Ageing is associated with impaired function and viability of EPCs leading to decrease of endothelial reparatory potential.44 On the other hand, use of statins may reverse the process by increasing the mobilization, and improving the viability, migratory and neovascularization capacity. In patients with stable CAD age is inversely correlated with the number and functional capacity of circulating EPCs; however, the mobilization of these cells in acute MI is not significantly related to age.45 Conversely, we found that mobilization of primitive VSELs in acute MI is reduced in older patients.27
Full size table
Full size table
In addition, several other, non-cardiovascular clinical situations may alter the number and properties of circulating BM-derived SPCs, which supports the theory that this mechanism is universally involved in tissue repair (Table 3).
Full size table
Mobilization of SPCs and clinical outcomes in acute coronary syndromes
In theory, the effectiveness of the mobilization reflects the intrinsic potential for tissue repair and could be a valuable prognostic marker. Observational studies suggest that mobilization of CD34+ cells is correlated with parameters of left ventricular function and remodeling 1 year after acute MI.23 In our study numbers of CD34+/CXCR4+ and CD34+/CD117+ in the early phase of acute MI showed positive correlation with left ventricular ejection fraction and negative correlation with NT-proBNP and cardiac necrosis markers. We showed that in patients with severely impaired left ventricular ejection fraction the mobilization of CD34+ cells and VSELs was significantly less effective than in patients with better contractility.46 The role of circulating CXCR4+ cells as a predictors of long-term improvement of left ventricular ejection fraction needs confirmation in larger groups of patients; however, the available data suggest that impaired mobilization of SPC might identify patients with more severe cardiac necrosis and dysfunction.27 It is unclear whether the inverse association between mobilization of BM cells and extent of myocardial infarct size is a result of more rapid engraftment of cells or signifies the impaired potential of myocardial healing. Data on the prognostic value of other populations of SPC in acute MI are conflicting.3, 8, 10 Moreover, not only the number of EPCs, but also their capability to differentiate into adult endothelial cells may affect functional improvement and infarct size reduction evidenced by nuclear scan, left ventricular ejection fraction salvage and favorable remodeling in patients with AMI.47 The mobilization of EPCs 24 h after acute MI showed significant positive correlation with extent of viable ischemic myocardium, but not with infarct size and microvascular obstruction. This supports the hypothesis that myocardial ischemia, and not necrosis, is a pivotal determinant of EPCs release from the BM.48 Also, the number of EPCs isolated from the arterial blood drawn from the coronary artery distally to the occlusion in acute MI was positively correlated with successful revascularization measured by myocardial blood flow.49 The generalized vascular injury in cardiovascular disease leads to a detachment of mature EC from the basement membrane and increase in the cellular turnover. One might hypothesize that release of BM-derived EPCs should be sufficient to replace the detached EC and restore the functionally competent endothelial layer. Therefore, measurement of these two populations should be considered a potential tool for risk stratification, because it reflects not only the endothelial injury but also individual physiological repair response.20 It seems logical to interpret differently the release of SPCs triggered by acute ischemic injury (for example, MI) and fluctuations in their numbers in stable conditions, and minor vascular trauma. Endovascular procedures, such as insertion of coronary catheter and percutaneous coronary intervention with implantation of stent, lead to local injury of endothelium, evidenced by release of both CEC as a direct result of injury and EPCs, which is most likely the reparatory response.50
Circulating BM cells and risk stratification in stable coronary heart disease and heart failure
Stable CAD represents a situation entirely different from acute MI in terms of BM cell mobilization. The number of cells constantly released might be considered to be the individual competence for endothelial repair to counterbalance the apoptosis and detachment of EC from the luminal surface of the artery. A prospective study of 519 patients with stable CAD demonstrated increased risk of CV associated with low number of circulating EPCs.51 Not only the number but also impaired function of EPCs displays negative correlation with CV risk.52 Hill et al. 18 showed inverse correlation between the circulating EPCs and Framingham risk score in subjects without CAD. Interestingly, the number of circulating EPC count was a good predictor of endothelium-dependent vasodilatation.18 Recently published data from analysis of more than 1100 patients showed that measurement of circulating CPCs may have additional prognostic value, especially in patients with high risk of cardiovascular events. Suppression of SPC mobilization in heart failure is particularly evident in more severe classes of cardiac dysfunction. Several studies showed that measurement of circulating EPCs might predict the outcome, including all-cause mortality, in this population of high-risk patients.53 Therefore, the enumeration of circulating EPCs may be used in risk stratification, because it seems to reflect the global burden of CV risk factors.54
Dysfunction of BM-derived cells
Even if the mobilization of progenitor cells is targeted at reparatory reaction, it is obviously not sufficient to rebuild the necrotic tissue. One of the reasons is a relatively low number of cells in comparison with the extent of necrotic cells within the heart. Also, the populations of mobilized cells might be dysfunctional. Schwartzenberg et al. 55 showed that in patients with ACS the number of apoptotic CD34+ Anexin V+ cells was significantly increased than in healthy controls and correlated with severity of coronary disease.
Notably, in addition to alterations in the number of circulating or mobilized stem/progenitor cells in cardiovascular disease, several recent studies have clearly suggested that there is a major alteration of the CV-repair function, that is, the capacity to promote repair of endothelial or cardiac injury, mediated by circulating or mobilized stem/progenitor cells in patients with cardiovascular risk factors or established CV disease. The vascular or cardiac repair capacity of circulating SPC cells has been studied in numerous in-vitro and in several in-vivo functional assays, that is, frequently involving transplantation of patient-derived stem/progenitor cells into immunodeficient animal models with either vascular or peripheral and cardiac ischemic injury. The impairment of the vascular, that is, endothelial, repair capacity of 'early EPCs' likely is an early event in the CV disease process, as suggested, for example, by a recent study in patients with prehypertension as their only CV risk factor, who already had a markedly impaired endothelial repair capacity of circulating 'early EPCs' that was largely mediated by paracrine factors. In this study, signs of aging, such as telomere shortening and increased galactosidase activity, were observed in patient-derived early EPCs.56 In patients with type-2 diabetes, several studies have shown an impaired functional activity of 'early EPCs', including a loss of the capacity to promote endothelial repair.57, 58 Interestingly, the mechanisms underlying the impaired endothelial repair function likely included a reduced endothelial No synthase-derived nitric oxide (NO) production of patient-derived cells.58 The aspect of an impaired CV repair function of circulating SPCs is also particularly relevant for autologous cell transplantation in patients with ischemic heart disease, where eligible patients are elderly, and frequently have several CV risk factors. Autologous patient-derived BM or circulating cells have therefore been evaluated in preclinical studies and in-vitro and in-vivo functional assays. BM-derived mononuclear cells isolated from patients with ischemic cardiomyopathy had an impaired pro-angiogenic capacity when transplanted into mice with hindlimb ischemia.59 Moreover, several factors, including age, anemia, renal failure, high levels of C-reactive protein or interleukin-6, have been observed to correlate with a poor angiogenic potency of BM-derived mononuclear cells isolated from patients when examined after transplantation into mice with hindlimb ischemia.60
In addition, the in-vitro migratory capacity of infused BMCs and CPCs at the time of intra-coronary injection was closely related to cell-dependent reduction in infarct size in patients after acute MI.61 In the same line, transplantation of BMCs with a high mitogenic capacity in vitro as determined by colony-forming units was associated with significantly reduced markers of heart failure, namely NT-proBNP and NT-proANP, and mortality within 3 months after injection.62 In addition, a recent study suggested the effect of an association between CV risk factors, in particular with an effect of active smoking, on the therapeutic benefit of intracoronary BMC transplantation to cardiac function, that is, patients who are active smokers may benefit less from intracoronary BMC injection as compared with non-smokers as observed in the BONAMI trial (clinicaltrials.gov: NCT00200707).63 These observations may help identify likely responders and exclude patients who are not likely to receive benefit from cardiac cell transplantation.
Physical exercise
Regular exercise is one of the most important issues in primary and secondary prevention of cardiovascular events. In patients with CAD, the increased oxygen demand and myocardial ischemia during a single episode of exercise coexist with release of VEGF and mobilization of EPCs. The number of EPCs increases as soon as 10 min after the exercise and reaches its maximum after 24–48 h in patients with peripheral vascular disease (PVD). Prolonged 4-week exercise program seems not only to prolong the mobilization of EPCs but also to improve their function. This suggests that even in patients with diffuse atherosclerosis and multiple risk factors some reparative potential dependent on circulating BM-derived EPCs is retained and that it can be enhanced in a most physiological way.36, 64 Additionally, both maximal and endurance exercise can influence the numbers of both HSC and EPCs in healthy subjects. In the marathon runners (endurance exercise) the number of EPCs increased while erythropoietic and granulocyte monocyte colonies decreased after the run. On the other hand the field test (maximal exercise) led to increase of CD34+ and EPCs, as well as both types of hematopoietic colonies.65 Regular physical exercise is recommended to patients after ACS to improve the outcome. The beneficial effects of such training might be related to the improved mobilization and functional capacity of CPCs, as shown in patients with acute MI who underwent a 3-week period of regular exercise. After the training period the number and migratory capacity of CPCs (CD34+/CD45+ and CD133+/CD45+) significantly increased.66 Physical exercise can reverse the dysfunction of circulating angiogenic cells in patients with chronic heart failure. After a single episode of treadmill exercise, the migration and SDF-1 levels were comparable to healthy subjects. It remains, however, to be proven that regular exercise will lead to a sustained functional improvement of circulating angiogenic cells.67
Future challenges
In our opinion, the lack of consensus on the identity and methodology of measurement of EPCs is the most important limitation of discussed studies. To validate the clinical use in risk stratification, standardized protocols need to be developed and 'normal' values established. Also, the protocols for stem cell measurements will need to be simplified to be introduced into clinical practice. The incremental prognostic value of EPCs beyond the traditional risk factors has to be proved. Also, to move the field forward, the contribution of circulating SPCs to endothelial and myocardial repair in humans needs to be proven. Better understanding of the stem cell mobilization and homing will pave the way to introduce new pharmacological agents, which would stimulate the release of most potent populations of cells to achieve better results.
Summary
Acute CV-ischemic episodes induce the rapid mobilization of BM-derived cells. Subpopulations of circulating cells may participate in myocardial and endothelial repair; however, the efficiency of this process in unknown. The number and function of these cells is impaired in patients with diabetes and other CV risk factors, but can be improved by physical exercise and use of statins. The mobilization of SPC in acute coronary syndromes and stable CAD seems to predict the clinical outcomes in selected groups of patients. Interpretation of the findings has to incorporate other factors that modulate the process of mobilization, such as coexisting diseases, age and medications, as well as the details of the assay protocols.
References
- 1
Wojakowski W, Kucia M, Kazmierski M, Ratajczak MZ, Tendera M . Circulating progenitor cells in stable coronary heart disease and acute coronary syndromes: relevant reparatory mechanism? Heart 2008; 94: 27–33.
Article CAS PubMed Google Scholar
- 2
Wollert KC, Drexler H . Clinical applications of stem cells for the heart. Circ Res 2005; 96: 151–163.
Article CAS PubMed Google Scholar
- 3
Shintani S, Murohara T, Ikeda H, Ueno T, Honma T, Katoh A et al. Mobilization of endothelial progenitor cells in patients with acute myocardial infarction. Circulation 2001; 103: 2776–2779.
Article CAS PubMed Google Scholar
- 4
Ingram DA, Caplice NM, Yoder MC . Unresolved questions, changing definitions, and novel paradigms for defining endothelial progenitor cells. Blood 2005; 106: 1525–1531.
Article CAS PubMed Google Scholar
- 5
Urbich C, Dimmeler S . Endothelial progenitor cells: characterization and role in vascular biology. Circ Res 2004; 95: 343–353.
Article CAS Google Scholar
- 6
Leor J, Marber M . Endothelial progenitors: a new Tower of Babel? J Am Coll Cardiol 2006; 48: 1588–1590.
Article PubMed Google Scholar
- 7
Steinmetz M, Nickenig G, Werner N . Endothelial-regenerating cells: an expanding universe. Hypertension 2010; 55: 593–599.
Article CAS PubMed Google Scholar
- 8
Massa M, Rosti V, Ferrario M, Campanelli R, Ramajoli I, Rosso R et al. Increased circulating hematopoietic and endothelial progenitor cells in the early phase of acute myocardial infarction. Blood 2005; 105: 199–206.
Article CAS PubMed Google Scholar
- 9
Brehm M, Ebner P, Picard F, Urbien R, Turan G, Strauer BE . Enhanced mobilization of CD34(+) progenitor cells expressing cell adhesion molecules in patients with STEMI. Clin Res Cardiol 2009; 98: 477–486.
Article CAS PubMed Google Scholar
- 10
Gaspardone A, Menghini F, Mazzuca V, Skossyreva O, Barbato G, de Fabritiis P . Progenitor cell mobilisation in patients with acute and chronic coronary artery disease. Heart 2006; 92: 253–254.
Article CAS PubMed PubMed Central Google Scholar
- 11
George J, Goldstein E, Abashidze S, Deutsch V, Shmilovich H, Finkelstein A et al. Circulating endothelial progenitor cells in patients with unstable angina: association with systemic inflammation. Eur Heart J 2004; 25: 1003–1008.
Article CAS PubMed Google Scholar
- 12
Asahara T, Murohara T, Sullivan A, Silver M, van der Zee R, Li T et al. Isolation of Putative Progenitor Endothelial Cells for Angiogenesis. Science 1997; 275: 964–966.
Article CAS PubMed Google Scholar
- 13
Peichev M, Naiyer AJ, Pereira D, Zhu Z, Lane WJ, Williams M et al. Expression of VEGFR-2 and AC133 by circulating human CD34(+) cells identifies a population of functional endothelial precursors. Blood 2000; 95: 952–958.
CAS PubMed Google Scholar
- 14
Rohde E, Malischnik C, Thaler D, Maierhofer T, Linkesch W, Lanzer G et al. Blood monocytes mimic endothelial progenitor cells. Stem cells (Dayton, OH) 2006; 24: 357–367.
Article Google Scholar
- 15
Elsheikh E, Uzunel M, He Z, Holgersson J, Nowak G, Sumitran-Holgersson S . Only a specific subset of human peripheral-blood monocytes has endothelial-like functional capacity. Blood 2005; 106: 2347–2355.
Article CAS PubMed Google Scholar
- 16
Krankel N, Katare RG, Siragusa M, Barcelos LS, Campagnolo P, Mangialardi G et al. Role of kinin B2 receptor signaling in the recruitment of circulating progenitor cells with neovascularization potential. Circ Res 2008; 103: 1335–1343.
Article CAS PubMed PubMed Central Google Scholar
- 17
Schmidt-Lucke C, Fichtlscherer S, Aicher A, Tschope C, Schultheiss HP, Zeiher AM et al. Quantification of circulating endothelial progenitor cells using the modified ISHAGE protocol. PLoS One 2010; 5: e13790.
Article CAS PubMed PubMed Central Google Scholar
- 18
Hill JM, Zalos G, Halcox JP, Schenke WH, Waclawiw MA, Quyyumi AA et al. Circulating endothelial progenitor cells, vascular function, and cardiovascular risk. N Engl J Med 2003; 348: 593–600.
Article PubMed PubMed Central Google Scholar
- 19
Povsic TJ, Zavodni KL, Kelly FL, Zhu S, Goldschmidt-Clermont PJ, Dong C et al. Circulating progenitor cells can be reliably identified on the basis of aldehyde dehydrogenase activity. J Am Coll Cardiol 2007; 50: 2243–2248.
Article CAS PubMed Google Scholar
- 20
Boos CJ, Lip GY, Blann AD . Circulating endothelial cells in cardiovascular disease. J Am Coll Cardiol 2006; 48: 1538–1547.
Article CAS PubMed Google Scholar
- 21
Fadini GP, de Kreutzenberg SV, Coracina A, Baesso I, Agostini C, Tiengo A et al. Circulating CD34+ cells, metabolic syndrome, and cardiovascular risk. Eur Heart J 2006; 27: 2247–2255.
Article CAS PubMed Google Scholar
- 22
Wojakowski W, Tendera M, Michalowska A, Majka M, Kucia M, Maslankiewicz K et al. Mobilization of CD34/CXCR4+, CD34/CD117+, c-met+ stem cells, and mononuclear cells expressing early cardiac, muscle, and endothelial markers into peripheral blood in patients with acute myocardial infarction. Circulation 2004; 110: 3213–3220.
Article CAS PubMed Google Scholar
- 23
Leone AM, Rutella S, Bonanno G, Abbate A, Rebuzzi AG, Giovannini S et al. Mobilization of bone marrow-derived stem cells after myocardial infarction and left ventricular function. Eur Heart J 2005; 26: 1196–1204.
Article PubMed Google Scholar
- 24
Zuba-Surma EK, Ratajczak MZ . Overview of very small embryonic-like stem cells (VSELs) and methodology of their identification and isolation by flow cytometric methods. Curr Protoc Cytom 2010, Chapter 9: Unit9, 29.
- 25
Kucia M, Reca R, Campbell FR, Zuba-Surma E, Majka M, Ratajczak J et al. A population of very small embryonic-like (VSEL) CXCR4(+)SSEA-1(+)Oct-4+ stem cells identified in adult bone marrow. Leukemia 2006; 20: 857–869.
Article CAS Google Scholar
- 26
Wojakowski W, Kucia M, Zuba-Surma E, Jadczyk T, Ksiazek B, Ratajczak MZ et al. Very small embryonic-like stem cells in cardiovascular repair. Pharmacol Ther 2011; 129: 21–28.
Article CAS PubMed Google Scholar
- 27
Wojakowski W, Tendera M, Kucia M, Zuba-Surma E, Paczkowska E, Ciosek J et al. Mobilization of bone marrow-derived Oct-4+ SSEA-4+ very small embryonic-like stem cells in patients with acute myocardial infarction. J Am Coll Cardiol 2009; 53: 1–9.
Article CAS PubMed PubMed Central Google Scholar
- 28
Wojakowski W, Tendera M, Kucia M, Zuba-Surma E, Milewski K, Wallace-Bradley D et al. Cardiomyocyte differentiation of bone marrow-derived Oct-4+CXCR4+SSEA-1+ very small embryonic-like stem cells. Int J Oncol 2010; 37: 237–247.
CAS PubMed Google Scholar
- 29
Wang Y, Johnsen HE, Mortensen S, Bindslev L, Ripa RS, Haack-Sorensen M et al. Changes in circulating mesenchymal stem cells, stem cell homing factor, and vascular growth factors in patients with acute ST elevation myocardial infarction treated with primary percutaneous coronary intervention. Heart 2006; 92: 768–774.
Article CAS PubMed Google Scholar
- 30
Iso Y, Spees JL, Sato T, Koba S, Kobayashi YYT et al. CD271 identifies human bone marrow stem/progenitor cells with a proangiogenic potential and circulating progenitor cells mobilized after acute myocardial infarction. J Am Coll Cardiol 2010; 55: A176.E167.
Article Google Scholar
- 31
Paczkowska E, Kucia M, Koziarska D, Halasa M, Safranow K, Masiuk M et al. Clinical evidence that very small embryonic-like stem cells are mobilized into peripheral blood in patients after stroke. Stroke 2009; 40: 1237–1244.
Article CAS Google Scholar
- 32
Chu K, Jung KH, Lee ST, Park HK, Sinn DI, Kim JM et al. Circulating endothelial progenitor cells as a new marker of endothelial dysfunction or repair in acute stroke. Stroke 2008; 39: 1441–1447.
Article CAS PubMed Google Scholar
- 33
Sandri M, Beck EB, Adams V, Gielen S, Lenk K, Hollriegel R et al. Maximal exercise, limb ischemia, and endothelial progenitor cells. Eur J Cardiovasc Prev Rehabil 2011; 18: 55–64.
Article PubMed Google Scholar
- 34
Kim SK, Pak HN, Park JH, Choi JI, Nam MH, Jo Y et al. Non-ischaemic titrated cardiac injury caused by radiofrequency catheter ablation of atrial fibrillation mobilizes CD34-positive mononuclear cells by non-stromal cell-derived factor-1alpha mechanism. Europace 2009; 11: 1024–1031.
Article PubMed Google Scholar
- 35
Stein A, Wessling G, Deisenhofer I, Busch G, Steppich B, Estner H et al. Systemic inflammatory changes after pulmonary vein radiofrequency ablation do not alter stem cell mobilization. Europace 2008; 10: 444–449.
Article PubMed Google Scholar
- 36
Adams V, Lenk K, Linke A, Lenz D, Erbs S, Sandri M et al. Increase of circulating endothelial progenitor cells in patients with coronary artery disease after exercise-induced ischemia. Arterioscler Thromb Vasc Biol 2004; 24: 684–690.
Article CAS PubMed Google Scholar
- 37
Theiss HD, David R, Engelmann MG, Barth A, Schotten K, Naebauer M et al. Circulation of CD34+ progenitor cell populations in patients with idiopathic dilated and ischaemic cardiomyopathy (DCM and ICM). Eur Heart J 2007; 28: 1258–1264.
Article PubMed Google Scholar
- 38
Kucia M, Dawn B, Hunt G, Guo Y, Wysoczynski M, Majka M et al. Cells expressing early cardiac markers reside in the bone marrow and are mobilized into the peripheral blood after myocardial infarction. Circ Res 2004; 95: 1191–1199.
Article CAS PubMed PubMed Central Google Scholar
- 39
Ratajczak MZ, Lee H, Wysoczynski M, Wan W, Marlicz W, Laughlin MJ et al. Novel insight into stem cell mobilization-plasma sphingosine-1-phosphate is a major chemoattractant that directs the egress of hematopoietic stem progenitor cells from the bone marrow and its level in peripheral blood increases during mobilization due to activation of complement cascade/membrane attack complex. Leukemia 2010; 24: 976–985.
CAS Article Google Scholar
- 40
Ratajczak MZ, Kim CH, Wojakowski W, Janowska-Wieczorek A, Kucia M, Ratajczak J . Innate immunity as orchestrator of stem cell mobilization. Leukemia 2010; 24: 1667–1675.
Article CAS Google Scholar
- 41
Nian M, Lee P, Khaper N, Liu P . Inflammatory cytokines and postmyocardial infarction remodeling. Circ Res 2004; 94: 1543–1553.
Article CAS PubMed Google Scholar
- 42
Kucia M, Wojakowski W, Reca R, Machalinski B, Gozdzik J, Majka M et al. The migration of bone marrow-derived non-hematopoietic tissue-committed stem cells is regulated in an SDF-1-, HGF-, and LIF-dependent manner. Arch Immunol Ther Exp (Warsz) 2006; 54: 121–135.
Article CAS Google Scholar
- 43
Atsuhiko Oikawa MS, Quaini F, Mangialardi G, Katare RG, Caporali A, van Buul JD et al. Diabetes Mellitus Induces Bone Marrow Microangiopathy. Arterioscler Thromb Vasc Biol 2010; 30: 498–508.
Article CAS PubMed Google Scholar
- 44
Heiss C, Keymel S, Niesler U, Ziemann J, Kelm M, Kalka C . Impaired progenitor cell activity in age-related endothelial dysfunction. J Am Coll Cardiol 2005; 45: 1441–1448.
Article CAS PubMed Google Scholar
- 45
Kondo T, Shintani S, Maeda K, Hayashi M, Inden Y, Numaguchi Y et al. The number and function of circulating CD34+CD133+ progenitor cells decreased in stable coronary artery disease but not in acute myocardial infarction. Heart Asia 2010; 2: 20–23.
Article PubMed PubMed Central Google Scholar
- 46
Wojakowski W, Tendera M, Zebzda A, Michalowska A, Majka M, Kucia M et al. Mobilization of CD34(+), CD117(+), CXCR4(+), c-met(+) stem cells is correlated with left ventricular ejection fraction and plasma NT-proBNP levels in patients with acute myocardial infarction. Eur Heart J 2006; 27: 283–289.
Article CAS PubMed Google Scholar
- 47
Numaguchi Y, Sone T, Okumura K, Ishii M, Morita Y, Kubota R et al. The impact of the capability of circulating progenitor cell to differentiate on myocardial salvage in patients with primary acute myocardial infarction. Circulation 2006; 114 (1 Suppl): I114–I119.
PubMed Google Scholar
- 48
Porto I, Leone AM, De Maria GL, Craig CH, Tritarelli A, Camaioni C et al. Are endothelial progenitor cells mobilized by myocardial ischemia or myocardial necrosis? A cardiac magnetic resonance study. Atherosclerosis 2011, (in press).
- 49
Porto I, Biasucci LM, De Maria GL, Di Vito L, Leone AM, Niccoli G et al. Increased levels of circulating endothelial progenitor cells in intracoronary blood of ST-elevation myocardial infarction patients correlate with microvascular damage. J Am Coll Cardiol 2010; 55: A187.E1751.
Article Google Scholar
- 50
Boos CJ, Balakrishnan B, Jessani S, Blann AD, Lip GY . Effects of percutaneous coronary intervention on peripheral venous blood circulating endothelial cells and plasma indices of endothelial damage/dysfunction. Chest 2007; 132: 1920–1926.
Article CAS PubMed Google Scholar
- 51
Werner N, Kosiol S, Schiegl T, Ahlers P, Walenta K, Link A et al. Circulating endothelial progenitor cells and cardiovascular outcomes. N Engl J Med 2005; 353: 999–1007.
Article CAS PubMed PubMed Central Google Scholar
- 52
Vasa M, Fichtlscherer S, Aicher A, Adler K, Urbich C, Martin H et al. Number and migratory activity of circulating endothelial progenitor cells inversely correlate with risk factors for coronary artery disease. Circ Res 2001; 89: E1–E7.
Article CAS PubMed Google Scholar
- 53
Michowitz Y, Goldstein E, Wexler D, Sheps D, Keren G, George J . Circulating endothelial progenitor cells and clinical outcome in patients with congestive heart failure. Heart 2007; 93: 1046–1050.
Article PubMed PubMed Central Google Scholar
- 54
Fadini GP, Maruyama S, Ozaki T, Taguchi A, Meigs J, Dimmeler S et al. Circulating progenitor cell count for cardiovascular risk stratification: a pooled analysis. PLoS One 2010; 5: e11488.
Article CAS PubMed PubMed Central Google Scholar
- 55
Schwartzenberg S, Deutsch V, Maysel-Auslender S, Kissil S, Keren G, George J . Circulating apoptotic progenitor cells: a novel biomarker in patients with acute coronary syndromes. Arterioscler Thromb Vasc Biol 2007; 27: e27–e31.
Article CAS PubMed Google Scholar
- 56
Giannotti G, Doerries C, Mocharla PS, Mueller MF, Bahlmann FH, Horvath T et al. Impaired Endothelial Repair Capacity of Early Endothelial Progenitor Cells in Prehypertension. Relation to Endothelial Dysfunction. Hypertension 2010; 55: 1389–1397.
Article CAS PubMed Google Scholar
- 57
Tepper OM, Galiano RD, Capla JM, Kalka C, Gagne PJ, Jacobowitz GR et al. Human endothelial progenitor cells from type II diabetics exhibit impaired proliferation, adhesion, and incorporation into vascular structures. Circulation 2002; 106: 2781–2786.
Article PubMed Google Scholar
- 58
Sorrentino SA, Bahlmann FH, Besler C, Muller M, Schulz S, Kirchhoff N et al. Oxidant stress impairs in vivo reendothelialization capacity of endothelial progenitor cells from patients with type 2 diabetes mellitus: restoration by the peroxisome proliferator-activated receptor-gamma agonist rosiglitazone. Circulation 2007; 116: 163–173.
Article CAS PubMed Google Scholar
- 59
Sasaki K, Heeschen C, Aicher A, Ziebart T, Honold J, Urbich C et al. Ex vivo pretreatment of bone marrow mononuclear cells with endothelial NO synthase enhancer AVE9488 enhances their functional activity for cell therapy. Proc Natl Acad Sci USA 2006; 103: 14537–14541.
Article CAS PubMed Google Scholar
- 60
Li TS, Kubo M, Ueda K, Murakami M, Ohshima M, Kobayashi T et al. Identification of risk factors related to poor angiogenic potency of bone marrow cells from different patients. Circulation 2009; 120 (11 Suppl): S255–S261.
Article PubMed Google Scholar
- 61
Britten MB, Abolmaali ND, Assmus B, Lehmann R, Honold J, Schmitt J et al. Infarct remodeling after intracoronary progenitor cell treatment in patients with acute myocardial infarction (TOPCARE-AMI): mechanistic insights from serial contrast-enhanced magnetic resonance imaging. Circulation 2003; 108: 2212–2218.
Article CAS PubMed Google Scholar
- 62
Assmus B, Fischer-Rasokat U, Honold J, Seeger FH, Fichtlscherer S, Tonn T et al. Transcoronary transplantation of functionally competent BMCs is associated with a decrease in natriuretic peptide serum levels and improved survival of patients with chronic postinfarction heart failure: results of the TOPCARE-CHD Registry. Circ Res 2007; 100: 1234–1241.
Article CAS PubMed Google Scholar
- 63
Roncalli J, Mouquet F, Piot C, Trochu JN, Le Corvoisier P, Neuder Y et al. Intracoronary autologous mononucleated bone marrow cell infusion for acute myocardial infarction: results of the randomized multicenter BONAMI trial. Eur Heart J 2010, (in press).
- 64
Sandri M, Adams V, Gielen S, Linke A, Lenk K, Krankel N et al. Effects of exercise and ischemia on mobilization and functional activation of blood-derived progenitor cells in patients with ischemic syndromes: results of 3 randomized studies. Circulation 2005; 111: 3391–3399.
Article PubMed Google Scholar
- 65
Bonsignore MR, Morici G, Riccioni R, Huertas A, Petrucci E, Veca M et al. Hemopoietic and angiogenetic progenitors in healthy athletes: different responses to endurance and maximal exercise. J Appl Physiol 2010; 109: 60–67.
Article CAS PubMed Google Scholar
- 66
Brehm M, Picard F, Ebner P, Turan G, Bolke E, Kostering M et al. Effects of exercise training on mobilization and functional activity of blood-derived progenitor cells in patients with acute myocardial infarction. Eur J Med Res 2009; 14: 393–405.
PubMed PubMed Central Google Scholar
- 67
Van Craenenbroeck EM, Beckers PJ, Possemiers NM, Wuyts K, Frederix G, Hoymans VY et al. Exercise acutely reverses dysfunction of circulating angiogenic cells in chronic heart failure. Eur Heart J 2010; 31: 1924–1934.
Article CAS PubMed Google Scholar
- 68
Urbich C, Dimmeler S . Risk factors for coronary artery disease, circulating endothelial progenitor cells, and the role of HMG-CoA reductase inhibitors. Kidney Int 2005; 67: 1672–1676.
Article CAS PubMed Google Scholar
- 69
Spyridopoulos I, Haendeler J, Urbich C, Brummendorf TH, Oh H, Schneider MD et al. Statins enhance migratory capacity by upregulation of the telomere repeat-binding factor TRF2 in endothelial progenitor cells. Circulation 2004; 110: 3136–3142.
Article CAS PubMed Google Scholar
- 70
Vasa M, Fichtlscherer S, Adler K, Aicher A, Martin H, Zeiher AM et al. Increase in circulating endothelial progenitor cells by statin therapy in patients with stable coronary artery disease. Circulation 2001; 103: 2885–2890.
Article CAS PubMed Google Scholar
- 71
Werner N, Nickenig G . Influence of cardiovascular risk factors on endothelial progenitor cells: limitations for therapy? Arterioscler Thromb Vasc Biol 2006; 26: 257–266.
Article CAS PubMed Google Scholar
- 72
Dzau VJ, Gnecchi M, Pachori AS, Morello F, Melo LG . Therapeutic potential of endothelial progenitor cells in cardiovascular diseases. Hypertension 2005; 46: 7–18.
Article CAS PubMed Google Scholar
- 73
Wojakowski W, Tendera M . Mobilization of bone marrow-derived progenitor cells in acute coronary syndromes. Folia Histochem Cytobiol 2005; 43: 229–232.
PubMed Google Scholar
- 74
Shin D, Hong SJ, Choi JH, Kim JH, Kim JS, Park JH et al. Red ginseng extracts improved coronary flow reserve and increased absolute numbers of various circulating angiogenic cells in patients with ST-elevation myocardial infarction. J Am Coll Cardiol 2010; 55: A100.E936.
Article Google Scholar
- 75
Heiss C, Jahn S, Taylor M, Real WM, Angeli FS, Wong ML et al. Improvement of endothelial function with dietary flavanols is associated with mobilization of circulating angiogenic cells in patients with coronary artery disease. J Am Coll Cardiol 2010; 56: 218–224.
Article CAS PubMed Google Scholar
- 76
DiFabio JM, Thomas GR, Zucco L, Kuliszewski MA, Bennett BM, Kutryk MJ et al. Nitroglycerin attenuates human endothelial progenitor cell differentiation, function, and survival. J Pharmacol Exp Ther 2006; 318: 117–123.
Article CAS PubMed Google Scholar
- 77
Fadini GP, Boscaro E, Albiero M, Menegazzo L, Frison V, de Kreutzenberg S et al. The oral dipeptidyl peptidase-4 inhibitor sitagliptin increases circulating endothelial progenitor cells in patients with type 2 diabetes: possible role of stromal-derived factor-1alpha. Diabetes Care 2010; 33: 1607–1609.
Article CAS PubMed PubMed Central Google Scholar
- 78
Greenbaum AM, Link DC . Mechanisms of G-CSF-mediated hematopoietic stem and progenitor mobilization. Leukemia 2011; 25: 211–217.
Article CAS Google Scholar
- 79
Jujo K, Hamada H, Iwakura A, Thorne T, Sekiguchi H, Clarke T et al. CXCR4 blockade augments bone marrow progenitor cell recruitment to the neovasculature and reduces mortality after myocardial infarction. Proc Natl Acad Sci USA 2010; 107: 11008–11013.
Article CAS PubMed Google Scholar
- 80
Hristov M, Erl W, Weber PC . Endothelial progenitor cells: mobilization, differentiation, and homing. Arterioscler Thromb Vasc Biol 2003; 23: 1185–1189.
Article CAS PubMed Google Scholar
- 81
Hristov M, Weber C . The therapeutic potential of progenitor cells in ischemic heart disease–Past, present and future. Basic Res Cardiol 2006; 101: 1–7.
Article PubMed Google Scholar
- 82
Hristov M, Weber C . Endothelial progenitor cells: characterization, pathophysiology, and possible clinical relevance. J Cell Mol Med 2004; 8: 498–508.
Article PubMed Google Scholar
- 83
Ruel M, Suuronen EJ, Song J, Kapila V, Gunning D, Waghray G et al. Effects of off-pump versus on-pump coronary artery bypass grafting on function and viability of circulating endothelial progenitor cells. J Thorac Cardiovasc Surg 2005; 130: 633–639.
Article PubMed Google Scholar
- 84
Chen JZ, Zhang FR, Tao QM, Wang XX, Zhu JH, Zhu JH . Number and activity of endothelial progenitor cells from peripheral blood in patients with hypercholesterolaemia. Clin Sci (Lond) 2004; 107: 273–280.
Article CAS Google Scholar
- 85
Kondo T, Hayashi M, Takeshita K, Numaguchi Y, Kobayashi K, Iino S et al. Smoking cessation rapidly increases circulating progenitor cells in peripheral blood in chronic smokers. Arterioscler Thromb Vasc Biol 2004; 24: 1442–1447.
Article CAS PubMed Google Scholar
- 86
Muller-Ehmsen J, Braun D, Schneider T, Pfister R, Worm N, Wielckens K et al. Decreased number of circulating progenitor cells in obesity: beneficial effects of weight reduction. Eur Heart J 2008; 29: 1560–1568.
Article PubMed Google Scholar
- 87
Fadini GP, Miorin M, Facco M, Bonamico S, Baesso I, Grego F et al. Circulating endothelial progenitor cells are reduced in peripheral vascular complications of type 2 diabetes mellitus. J Am Coll Cardiol 2005; 45: 1449–1457.
Article CAS PubMed Google Scholar
- 88
Fadini GP, Boscaro E, de Kreutzenberg S, Agostini C, Seeger F, Dimmeler S et al. Time course and mechanisms of circulating progenitor cell reduction in the natural history of type 2 diabetes. Diabetes Care 2010; 33: 1097–1102.
Article PubMed PubMed Central Google Scholar
- 89
Valgimigli M, Rigolin GM, Fucili A, Porta MD, Soukhomovskaia O, Malagutti P et al. CD34+ and endothelial progenitor cells in patients with various degrees of congestive heart failure. Circulation 2004; 110: 1209–1212.
Article CAS PubMed Google Scholar
- 90
Matsumoto Y, Adams V, Walther C, Kleinecke C, Brugger P, Linke A et al. Reduced number and function of endothelial progenitor cells in patients with aortic valve stenosis: a novel concept for valvular endothelial cell repair. Eur Heart J 2009; 30: 346–355.
Article PubMed Google Scholar
- 91
Jickling G, Salam A, Mohammad A, Hussain MS, Scozzafava J, Nasser AM et al. Circulating endothelial progenitor cells and age-related white matter changes. Stroke 2009; 40: 3191–3196.
Article PubMed Google Scholar
- 92
Asosingh K, Aldred MA, Vasanji A, Drazba J, Sharp J, Farver C et al. Circulating angiogenic precursors in idiopathic pulmonary arterial hypertension. Am J Pathol 2008; 172: 615–627.
Article CAS PubMed PubMed Central Google Scholar
- 93
Eizawa T, Ikeda U, Murakami Y, Matsui K, Yoshioka T, Suzuki C et al. Increase in circulating endothelial progenitor cells after aortic aneurysm repair. Heart Vessels 2004; 19: 107–110.
Article PubMed Google Scholar
- 94
Gaspardone A, De Fabritiis P, Scaffa R, Nardi P, Palombi F, Versaci F et al. Stem cell mobilization after coronary artery bypass grafting]. Ital Heart J Suppl 2004; 5 (1 Suppl): 23–28.
PubMed Google Scholar
- 95
Mieno S, Ramlawi B, Boodhwani M, Clements RT, Minamimura K, Maki T et al. Role of Stromal-Derived Factor-1{alpha} in the Induction of Circulating CD34+CXCR4+ Progenitor Cells After Cardiac Surgery. Circulation 2006; 114 (1 Suppl): 186–192.
Article CAS Google Scholar
- 96
Simper D, Wang S, Deb A, Holmes D, McGregor C, Frantz R et al. Endothelial progenitor cells are decreased in blood of cardiac allograft patients with vasculopathy and endothelial cells of noncardiac origin are enriched in transplant atherosclerosis. Circulation 2003; 108: 143–149.
Article PubMed Google Scholar
- 97
Sugawara J, Mitsui-Saito M, Hoshiai T, Hayashi C, Kimura Y, Okamura K . Circulating endothelial progenitor cells during human pregnancy. J Clin Endocrinol Metab 2005; 90: 1845–1848.
Article CAS PubMed Google Scholar
- 98
Sugawara J, Mitsui-Saito M, Hayashi C, Hoshiai T, Senoo M, Chisaka H et al. Decrease and senescence of endothelial progenitor cells in patients with preeclampsia. J Clin Endocrinol Metab 2005; 90: 5329–5332.
Article CAS PubMed Google Scholar
- 99
Choi JH, Kim KL, Huh W, Kim B, Byun J, Suh W et al. Decreased number and impaired angiogenic function of endothelial progenitor cells in patients with chronic renal failure. Arterioscler Thromb Vasc Biol 2004; 24: 1246–1252.
Article CAS PubMed Google Scholar
- 100
Eizawa T, Murakami Y, Matsui K, Takahashi M, Muroi K, Amemiya M et al. Circulating endothelial progenitor cells are reduced in hemodialysis patients. Curr Med Res Opin 2003; 19: 627–633.
Article PubMed Google Scholar
- 101
Herbrig K, Pistrosch F, Foerster S, Gross P . Endothelial progenitor cells in chronic renal insufficiency. Kidney Blood Press Res 2006; 29: 24–31.
Article PubMed Google Scholar
- 102
Surdacki A, Marewicz E, Wieteska E, Szastak G, Rakowski T, Wieczorek-Surdacka E et al. Association between endothelial progenitor cell depletion in blood and mild-to-moderate renal insufficiency in stable angina. Nephrol Dial Transplant 2008; 23: 2265–2273.
Article CAS PubMed Google Scholar
- 103
Pirro M, Schillaci G, Romagno PF, Mannarino MR, Bagaglia F, Razzi R et al. Influence of Short-term Rosuvastatin Therapy on Endothelial Progenitor Cells and Endothelial Function. J Cardiovasc Pharmacol Ther 2009; 14: 14–21.
Article CAS PubMed Google Scholar
- 104
Liu X, Li Y, Liu Y, Luo Y, Wang D, Annex BH et al. Endothelial progenitor cells (EPCs) mobilized and activated by neurotrophic factors may contribute to pathologic neovascularization in diabetic retinopathy. Am J Pathol 2010; 176: 504–515.
Article CAS PubMed PubMed Central Google Scholar
- 105
Grisar J, Aletaha D, Steiner CW, Kapral T, Steiner S, Seidinger D et al. Depletion of endothelial progenitor cells in the peripheral blood of patients with rheumatoid arthritis. Circulation 2005; 111: 204–211.
Article PubMed Google Scholar
- 106
Herbrig K, Haensel S, Oelschlaegel U, Pistrosch F, Foerster S, Passauer J . Endothelial dysfunction in patients with rheumatoid arthritis is associated with a reduced number and impaired function of endothelial progenitor cells. Ann Rheum Dis 2006; 65: 157–163.
Article CAS PubMed Google Scholar
- 107
Yamada M, Kubo H, Ishizawa K, Kobayashi S, Shinkawa M, Sasaki H . Increased circulating endothelial progenitor cells in patients with bacterial pneumonia: evidence that bone marrow derived cells contribute to lung repair. Thorax 2005; 60: 410–413.
Article CAS PubMed PubMed Central Google Scholar
- 108
Fadini GP, Schiavon M, Cantini M, Baesso I, Facco M, Miorin M et al. Circulating progenitor cells are reduced in patients with severe lung disease. Stem cells (Dayton, OH) 2006; 24: 1806–1813.
Article Google Scholar
- 109
Palange P, Testa U, Huertas A, Calabro L, Antonucci R, Petrucci E et al. Circulating haemopoietic and endothelial progenitor cells are decreased in COPD. Eur Respir J 2006; 27: 529–541.
Article CAS PubMed Google Scholar
- 110
Lee ST, Chu K, Jung KH, Park HK, Kim DH, Bahn JJ et al. Reduced circulating angiogenic cells in Alzheimer disease. Neurology 2009; 72: 1858–1863.
Article PubMed Google Scholar
- 111
Dome B, Timar J, Dobos J, Meszaros L, Raso E, Paku S et al. Identification and clinical significance of circulating endothelial progenitor cells in human non-small cell lung cancer. Cancer Res 2006; 66: 7341–7347.
Article CAS PubMed Google Scholar
- 112
Shakoor SK, Aldibbiat A, Ingoe LE, Campbell SC, Sibal L, Shaw J et al. Endothelial progenitor cells in subclinical hypothyroidism: the effect of thyroid hormone replacement therapy. J Clin Endocrinol Metab 2010; 95: 319–322.
Article CAS PubMed Google Scholar
- 113
Brunner S, Theiss HD, Murr A, Negele T, Franz WM . Primary hyperparathyroidism is associated with increased circulating bone marrow-derived progenitor cells. Am J Physiol Endocrinol Metab 2007; 293: E1670–E1675.
Article CAS PubMed Google Scholar
- 114
Padfield GJ, Tura O, Haeck MLA, Short A, Freyer E, Barclay GR et al. Circulating endothelial progenitor cells are not affected by acute systemic inflammation. Am J Physiol Heart Circ Physiol 2010; 298: H2054–H2061.
Article CAS PubMed PubMed Central Google Scholar
- 115
Thill M, Strunnikova NV, Berna MJ, Gordiyenko N, Schmid K, Cousins SW et al. Late outgrowth endothelial progenitor cells in patients with age-related macular degeneration. Invest Ophthalmol Vis Sci 2008; 49: 2696–2708.
Article PubMed Google Scholar
Download references
Acknowledgements
This study was funded by the European Union structural funds—Innovative Economy Operational Programme, Grant No. POIG 01.02-00-109/09 'Innovative methods of stem cells applications in medicine', Polish Ministry of Science and Higher Education grants 0651/P01/2007/32 and 2422/P01/2007/32, and the Servier Research Grant.
Ethics declarations
Competing interests
The authors declare no conflict of interest.
Rights and permissions
About this article
Cite this article
Wojakowski, W., Landmesser, U., Bachowski, R. et al. Mobilization of stem and progenitor cells in cardiovascular diseases. Leukemia 26, 23–33 (2012). https://doi.org/10.1038/leu.2011.184
Download citation
-
Received:
-
Revised:
-
Accepted:
-
Published:
-
Issue Date:
-
DOI : https://doi.org/10.1038/leu.2011.184
Keywords
- mobilization
- stem cells
- endothelial progenitor cells
- very small embryonic-like cells
- myocardial infarction
Further reading
-
Role of stem cell mobilization in the treatment of ischemic diseases
Archives of Pharmacal Research (2019)
-
Transforming growth factor-β in stem cells and tissue homeostasis
Bone Research (2018)
-
Stem Cell Homing: a Potential Therapeutic Strategy Unproven for Treatment of Myocardial Injury
Journal of Cardiovascular Translational Research (2018)
-
Extracellular molecules in hematopoietic stem cell mobilisation
International Journal of Hematology (2017)
-
A novel view of the adult bone marrow stem cell hierarchy and stem cell trafficking
Leukemia (2015)
Chapter 25 Section 1 Guided Reading and Review Mobilization
Source: https://www.nature.com/articles/leu2011184
Belum ada Komentar untuk "Chapter 25 Section 1 Guided Reading and Review Mobilization"
Posting Komentar